Keywords: TBI, neuroinflammation, neurodegeneration, secondary injury
Introduction | Immune response post TBI
Traumatic brain injury (TBI) is one of the most complex and heterogeneous pathologies affecting the brain due to the multitude of mechanisms, severity, and characteristics of the affected patient (age, genetics, sex), primary and secondary lesions, as well as medical management. All these are increasing the difficulty of implementing effective treatments, making TBI a leading cause of patient mortality and disability on a global level [1,2].
In addition, although the direct cause and primary lesion of TBI are mostly known, frequently representing an easy step in the diagnosis and treatment, the management of the post-traumatic secondary lesions due to the triggering of a complex biochemical cascade remains a difficult task. Moreover, the post-TBI outcomes are also influenced by the presence of associated injuries (e.g., dynamical changes of the intracranial fluids, hypoxemia, or hypotension).
All things considered, the treatment and management of TBI aimed at improving outcomes are lacking, thus highlighting the need for a better understanding of the pathogenetic mechanisms, including primary and secondary injuries, to be able to address these adequately [1–3].
Primary injury and secondary injury in TBI
The primary injury is considered the insult taking place at the moment of the TBI, directly correlated with the cause and leading to straight harm of the nervous tissue (Figure 1).
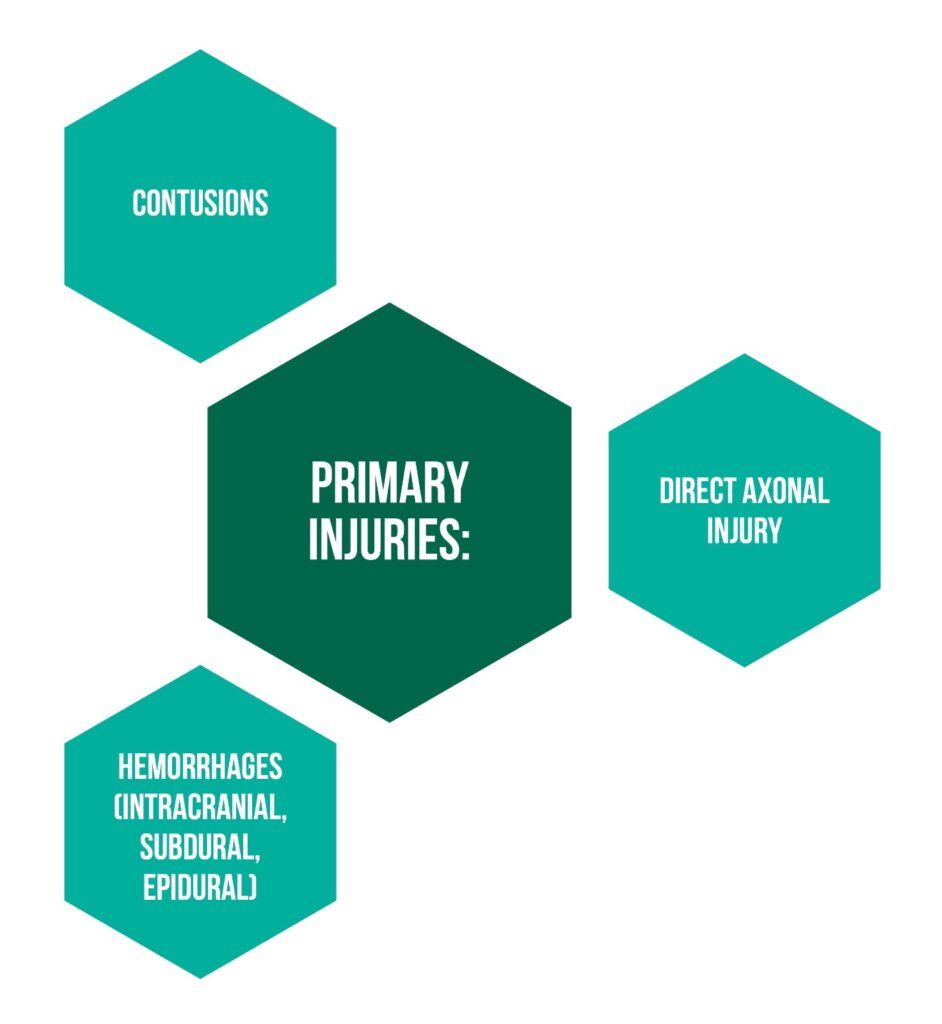
The secondary injuries, as mentioned above, are arbitrated by several cellular pathways (excitotoxicity, generation of free radicals, and neuroinflammatory reactions). Considering that the injury to neuronal cells is produced by the generation of free radicals affecting proteins and phospholipid membranes and toxic excitation due to excess glutamate, the immune-modulated neuroinflammatory response on a local and systemic level is a major topic of interest to be investigated. Even more so considering the proposed idea that modulation might influence the clinical outcomes after TBI [1].
The immune response after TBI is considered to start in the first minute post-injury leading, over time, to the activation of an inflammatory cascade with positive and negative effects, thus making the simple targeting of the immune response after TBI a way of improving clinical outcomes ineffective.
Jassam et al. proposed in their 2017 study a new stimulus- and disease-specific depiction of the natural immune responses based on transcriptomic and proteomic characterization, in contrast to the M1/M2 categorization, emphasizing the positive and negative consequences of the immunological response, as well as the missing information regarding the temporal impact of TBI-type [1].
Types of Immune Responses
The immune system of human beings, with its roles in defense and healing processes, is classified as “innate” and “adaptive” immunity (Figure 2).
The effector cells of the innate immune response can relate with Toll-like receptors (TLRs), nucleotide-binding oligomerization domain (NOD)-like receptors (NLRs), and scavenger receptors to ensure identification of pathogens and danger signals that lead to an increased “innate immune response” with the help of chemokines and cytokines that influence the recruitment of other cells and local and distal signaling. The various roles of the complement contribute to the innate immune response (pro-inflammatory signaling, marking, and forming of complexes used for damaging cells). Overall, the innate response leads to a reduced progression of pathogens and participates in healing and remodeling processes while preparing the scene for the more adapted immune response, namely the “adaptive” response [1–4].
The “adaptive” immune response is based on the activation and expansion of lymphocytes T and B. While CD8 and CD4 T lymphocytes can identify antigens and have regulatory, helper, or cytotoxic functions, B lymphocytes have the function of creating immunoglobulins and activation of T cells. Noteworthy is the ability of the “adaptive” response to lead to autoimmune responses leading either to disease or (if transient) to benefits [1].
The importance of TBI models for the research of fundamental inflammatory mechanisms
TBI is a heterogeneous disease with various causes leading to different types of injury (blast, concussion, contusion) that, in turn, lead to a wide array of pathological changes.
Between contusion and concussion, several differences were highlighted:
- the different mechanisms of trauma used in models
- the structural damage in contusion versus the more functional damage in concussion
- the different pathological responses (increased tissue inflammatory response for contusion versus the limited inflammation, but meningeal vascular leakage and gliosis for concussion).
Furthermore, these differences were emphasized by the fact that despite influencing the same inflammatory pathways, different responses were obtained for concussion and contusion. Similarly, in the case of a blast, the consequences differ regarding inflammation and axonal lesions or neurodegeneration. Consequently, the authors covered concussion and contusion in their research with well-defined specifications of the respective results [1].
The post-TBI inflammatory process and several of its significant components
Post-TBI, the inflammatory cascade is started following the interactivity between molecules localized in the lesioned or infected tissue (damage and pathogen-associated molecular patterns -DAMPs and pathogen-associated molecular patterns-PAMPs) and receptors indicating peril or danger (TLRs, NLRs, scavenger receptors).
The TLR4 that interacts with endogenous proteins (HMGB1, HSPs), lipopolysaccharides, and lipoproteins is increased following TBI in several types of cells (immune, neural), leading further to a cellular response involving the use of kinases that induce pathways that are increasing the appearance of TNF-alpha and IL-6 (inflammatory cytokines). Research has shown that on a functional level, TLR4 deficiency in mice has led to reduced cerebral injuries and the appearance of inflammatory markers and that an edema-reduction secondary to the use of TLR4 blocker leads to several modifications, including the blockage of IL-6 release via HMGB-1. HMGB-1 (having a dual function) is released by immune cells, and its connection to TLR4/RAGE initiates the inflammatory response.
Both HMBG-1 and RAGE are present post-TBI (animals and humans), their inhibition leading to a reduction in the destruction of the blood-brain barrier, inflammation, and pulmonary dysfunction in the context of contusion [1].
The expression of HSPs is enhanced by injuries and healing processes of lesions, having a double effect on the inflammation process, with enhancing and diminishing effects. Furthermore, it increases the immune reaction involved in antigen presentation and decreases lethality by reducing IL-6, nitric oxide, and TNF-alpha. In CCI-TBI, HSP70 led to a diminished brain lesion, hemorrhage, and increase of metalloproteinases, while its deficiency led to contrary consequences. Similarly, deficient HSP110 led to contrary consequences. Altogether, HSP70 and HSP110 could improve the outcomes of patients, and HSP might represent targets for the development of post-contusion TBI. Further research has shown that using an HSP90 antagonist leads to an increase in HSP70, reduced brain injury, and improved function [1].
Scavenger receptors (SCARF1, CD36, MEGF10) are needed for DAMP signaling in conjunction with TLRs. They are involved in the clearance of apoptotic cells and debris from the central nervous system, making them a possible target for patients with Alzheimer’s disease. However, there is still an information gap regarding TBI. Overall, in the context of contusion, the peril signals and their potential receptors have been studied on models, but the research on concussion is still presenting certain gaps.
Purinergic receptors have significant involvement in sterile injury reactions. The damaged tissue releases ATP (alarmin-like role), leading to the activation of the immunity response. The enzymes -convert ATP to ADP, and further adenosine is followed by purinergic receptor binding leading to a variety of responses depending on several aspects (the pattern of receptor`s expression, nature of lesion, period post-injury, and characteristics of the present immune cells).
The signals in these types of receptors have various roles:
- regulate the function in microglial cells after TBI (antagonizing some receptors leads to more damage)
- increase the recruitment of neutrophils to the lesion`s localization; this was shown in a focal head injury model, where the cell recruitment happened at the meningeal level, and the purinergic antagonization led to increased meningeal damage, thus revealing that promoting immunological purinergic signaling could have neuroprotective properties in the early stage after focal TBI
- the stimulation of a purinergic receptor (P2RY1) on astrocytes in a closed TBI model has led to a decrease in edema, neuronal swelling, and secondary gliosis)
- addon to activation of inflammasomes by the extracellular ATP activating purinergic receptors present on astrocytes and microglia (NLRP3 inflammasome activation) [1].
Inflammasomes also have a significant role in the induction of sterile immune responses post-TBI via the production of mature IL-1 beta and IL-18. The production follows the inflammasomes-induced transformation of procaspase-1 into caspase -1, where inflammasomes (macromolecular complexes) are made from NLR and non-NLR proteins (NLR being cytosolic receptors for DAMPs and PAMPs).
They can be found in a variety of cell types at the central nervous system level, and their activation would lead to the production of pro-inflammatory cytokines, making some of the protein possible markers of inflammation for TBI, even more so since some of the inflammasomes-included proteins have been identified in the cerebrospinal fluid (moderate and severe TBI) and correlated with unfavorable outcomes. Studies have shown different results regarding the activation of inflammasomes prompting the need for further research in order to establish the significance of the differences in the used TBI models, if the activation is pathologically uniform, and if the effects are cell-specific [1,4].
The guidance of the immune response (from development to function) is done with the help of acute inflammatory gene expression following the identification of DAMPs and alarmins. A 2012 study comparing the inflammatory gene expression in whole brain tissue secondary to craniotomy and craniotomy combined with cerebral contusion afterward showed a similar reaction, with genes being overexpressed (cytokine signaling, chemotaxis, antigen presentation, phagocytosis, activation of astrocytes) in both situations.
Contrasting was the nether extent and duration of inflammatory gene expression in the case of craniotomy (based on whole brain analysis, not cell analysis). Additionally, by comparing brain injury due to fluid percussion (as a mechanism for inflicting injury) and CCI, almost 90% of the observed mRNA that was regulated in a different way after mild FPI was also affected in CCI, thus raising the possibility of a link between severity and size of TBI, and the length and magnitude of inflammation.
Another important aspect regarding the inflammatory gene expression addresses the suffered transformations in time since studies have shown that inflammatory modifications can appear besides at the site of injury, also in the distality (including contralateral to the lesion). This might represent an explanation for part of post-TBI sequelae.
Lastly, two gaps would need to be further researched: the gene expression of single cells using newer methodologies (e.g., RNA-sequencing) as well as the identification of patterns of inflammatory gene expression and cell infiltration for all used TBI models in order to identify the one hand particular inflammatory characteristics and on the other hand, profiles that could have in some of the used models dual action that changes with time (beneficial and detrimental actions) [1].
The TBI biomarkers are showcased in Figure 3.
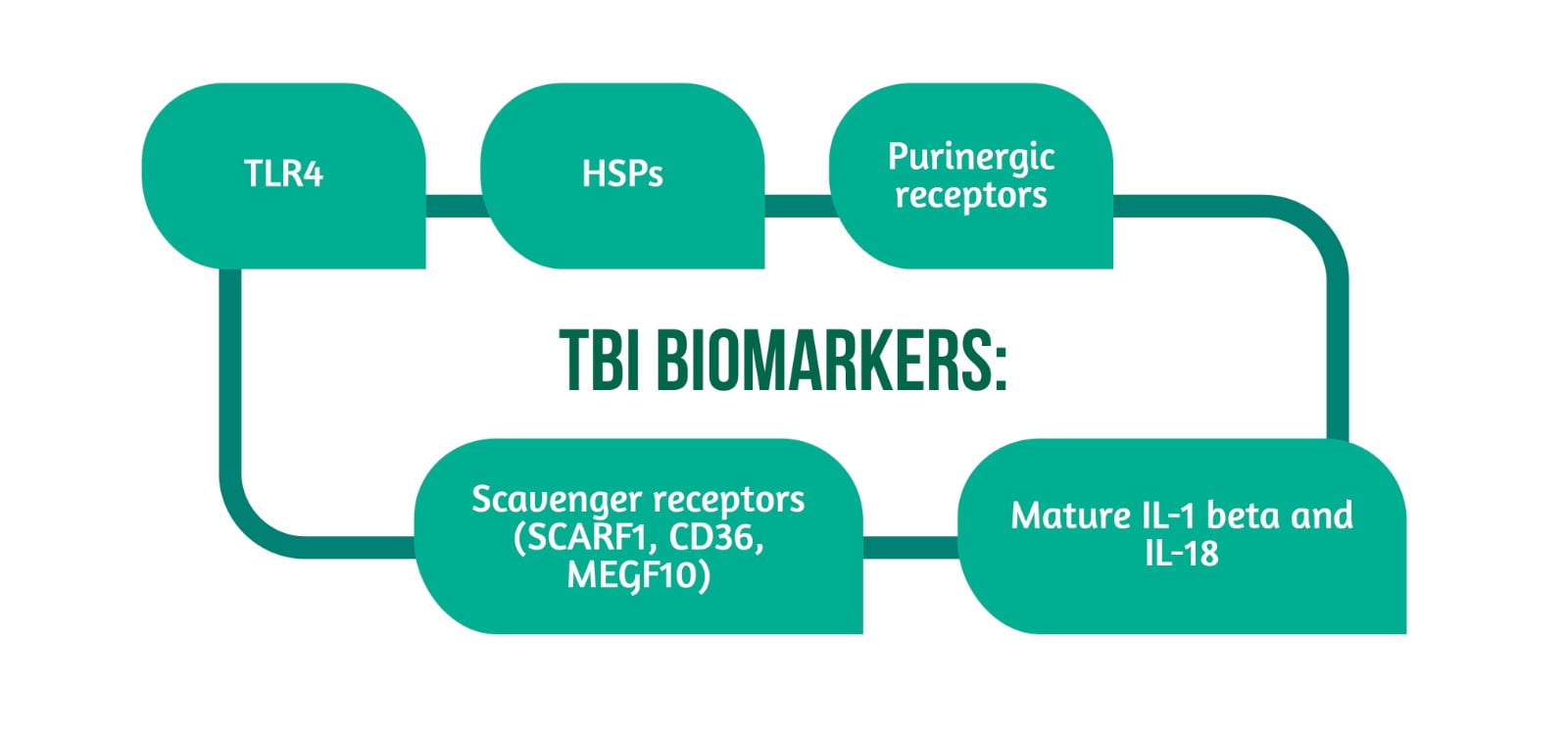
The role of Microglia in TBI
Microglia are cells derived from primitive macrophages during embryonic development that can be found at the CNS level. Due to gene expression, they have significant roles in the first minutes after focal TBI (although imagery studies have shown a duration of their activity up to several years post-TBI): screening and identification of inflammatory signs, phagocytosis of injured cells, assisting neuronal survival as well as boosting the modulation of activity-dependent synapses. These cells will extend several processes to the damaged site.
Post-concussion (at acute and chronic times), despite lacking important structural damage (e.g., acute cell death, hemorrhage, edema, etc.), both important microgliosis and astrocytosis were identified, while post-blast injuries microglial activation was identified.
The role of microglia post-TBI is still open to debate, considering its neurotoxic and neuroprotective characteristics during the multiphasic response (including structural modification, electrophysiological modifications, proliferation, relocation, distribution of cytokines/chemokines, and phagocytosis).
A main aspect regarding the effects of microglia activation should be time and context-based. Although some studies showed the main role of microglia to be a neurotoxic one, the neuroprotective one cannot be disputed, considering their involvement in the uncluttering of dead cells, the conservation of the blood-brain barrier (BBB) (namely the vasculature and glial limitans) together with neurotrophins release, all of these representing pillars for neural remodeling.
Post focal cortical TBI, the early structural changes of the resting microglia happen due to signaling in purinergic (P2Y6, P2X4, P2Y12) and tyrosine kinases (Mer, Tyro3, Axl) receptors, with studies showing that a barrier affecting the signaling leads to limited extension and conversion to the site of injury of microglia.
The response in the early period of microglia is critical for maintaining the conservation of structures of the BBB and phagocytosis (with P2Y6 activated by UDP facilitating it and increasing the mobility of microglia). Additionally, imagery-based studies have identified major microglia phagocytic networks post mild focal TBI at the glial limitans level, having maintenance and clean-up roles.
Followingly, antagonizing these early purinergic receptor microglial reactions increased brain injuries. Considering the proliferation of microglia cells (24h-weeks post TBI, in accordance with the type of lesion) secondary to released factors by the lesioned tissue, P2X7 (purinergic receptor) might potentially have an aiding role, while TGF-beta 1 cytokine and inhibitor role. Nevertheless, more research is needed for the exact identification of the contributive value of the proliferation of microglia and TGF-beta 1 for TBIs.
In addition, other roles of the microglia (detection of released DAMPs from injured cells, reactions to a variety of elements, including cytokines, glutamate, ions, neurotrophic factors, production of several inflammatory mediators, like Il-1beta, Il-12 or Il-6, metalloproteinases, reactive oxygen species) underline the importance of the contextuality for microglia, since this determines its beneficial (restorative) or detrimental role. Thus, since the immunological contribution depends on the state of activation, the severity of the lesion, the type of immune infiltrates, and the relation to other cells, which is time-changing, no definite statements can be made on microglia post-TBI.
Some studies attempted a therapeutic manipulation of microglia using minocycline, but the result was contrasting due to the failure of function-modulation of microglia and pleiotropic functions of minocycline, emphasizing the need for the use of genetic tools in future research to establish the role of microglia in brain injury (targeted deletion of specific genes in microglia or TBI pathogenetic inflammatory genes) as well as the influence of anti-inflammatory cytokines on microglial functions during the various phases of TBI.
Lastly, several studies have outlined the importance of gender differences in TBI, considering the identified variations of the inflammatory responses for mild to moderate CCI and moderate to severe CCI over time in males and females.
Additionally, the microglial function is affected by age, the aged microglia having fewer ramifications and purinergic response in contrast to young ones, making this also a subject for further research [1–4].
The polarization of microglia
Myeloid cells, including microglia, macrophages, and monocytes, are stimulated by individual cytokines (e.g., Il-6, Il-10) in vitro, followed by a gene expression measurement. This has led to the M1 and M2 polarization states classification. In contrast, in vivo, several factors and interactions influence the polarization of myeloid cells, ranging from the maturity of cells to up or down-regulated cytokine to adhesion molecules, making the reaction to single cytokines inadequate. Thus, despite identified differences and overlaps regarding functions, the M1 and M2 classification does not mirror the in vivo post-TBI different functions of microglia.
Even more, considering the variability of the microglial functions that can appear in the same tissue, a small gene analysis cannot reflect this aspect. The complexity and variability of the microglial polarization could be illustrated by using RNA-sequencing of single cells, thus identifying their profile during TBI. The results of a study investigating M1 markers of microglia in relation to aging emphasized the heterogeneity and complexity of microglial polarization during this physiological process, with some markers being upregulated, unchanged, or downregulated [1–4].
The role of Astrocytes regarding TBI
Astrocytes have several significant functions at the CNS level. They form the glia limitans, thus representing the barrier between CNS and vasculature, perivascular spaces and meninges, and a “gate” controlling the blood flow of immune cells. Recruitment-signaling of other immune cells and initiation of cytotoxic processes appear secondary to the transmembrane flux of ions (potassium efflux, calcium influx, ATP, and glutamate secretion) and by activated mechanoreceptors (by mechanical shear force).
Furthermore, reactive gliosis promotes the formation of a scar, thus limiting the damage and inflammation locally while also contributing to BBB restoration and maintenance of homeostasis (via nutritional support). Moreover, activated astrocytes play a role in inflammation via NF-kB pathway activation by chemokines/cytokines secretion, increased phagocytosis, and secretion of matrix metalloproteinase 9 (MMP-9). They also have correlated functions with other cells, including microglia, releasing, for example, various growth factors with healing function [1, 5].
The part of the post-TBI inflammatory response addressed in this first part of this digest, covering the research made on contusion and concussion in animal models, emphasized the post-inflammatory process and some of its components while highlighting, on the one hand, the importance of the “innate” and “adaptive” immunological response and on the other hand, possible targets for neuromodulation that could lead to reduced harmful processes. The above-mentioned examples emphasized consequences following the interactivity between molecules localized in the lesioned or infected tissue and the receptors indicating peril or danger.
A TLR deficiency led in animal models to reduced cerebral injury and inflammation, while a reduction also reduced lesional processes. HSP with dual action on the inflammatory processes has shown beneficiary activity – namely of HSP-70 and HSP-110, while the reduction of HSP-90 led to improved outcomes post-TBI. Scavenger receptors could represent a target for Alzheimer’s disease. Still, for contusion, the peril signals and their potential receptors have been studied on models, while the research on concussion is still lacking.
Furthermore, the purinergic receptors have shown various roles in the inflammatory process. At the same time, the inflammasomes, by producing pro-inflammatory cytokines, were correlated with worse outcomes, and their presence in CSF might be a marker of inflammation for TBI. Studies on inflammatory gene expression revealed several changes after TBI, one important aspect being the identification of inflammatory modifications besides the injury site prompting a possible explanation for post-TBI sequelae. Lastly, gaps regarding the identification of patterns of inflammatory gene expression and cell infiltration for all used TBI models remain to be studied to allow further identification of particular inflammatory characteristics and profiles that could have dual action that changes with time (beneficial and detrimental).
The role of the microglia in TBI is still to be determined, considering the dual neurotoxic and protective properties during the multiphase response post-TBI. The context and time of action are of essential importance, and the variations regarding the inflammatory response over time in genders and the influence of age on the microglia are also noteworthy. Furthermore, the M1 and M2 classification does not mirror the in vivo post-TBI different functions of the microglia. The roles of astrocytes are various, some of which are the process of scar-forming, part of the blood-brain barrier, and recruitment-signaling.
Continuing the description of this complex mechanism post-TBI, a future article will address the innate immune response after TBI, the inflammatory proteins, cytokines, metalloproteinases, the role of adaptive immunity in TBI, as well as the chronic inflammation post-TBI concluding with an emphasis on the critical significance of the inflammatory immune response post-TBI with its beneficial and detrimental elements, as well as proposed new methods and highlighting existing gaps that need to be addressed [1–5].
For more information about the impact of TBI visit:
- Assessment of Quality of Life after TBI using QOLIBRI-OS
- Can TBI affect taste and smell?
- TBI Outcomes in Europe
We kindly invite you to browse our Interview category https://brain-amn.org/category/interviews/. You will surely find a cluster of informative discussions with different specialists in the field of neurotrauma.
Bibliography
- Jassam YN, Izzy S, Whalen M, McGavern DB, El Khoury J. Neuroimmunology of Traumatic Brain Injury: Time for a Paradigm Shift. Neuron. 2017;95(6):1246-1265. doi:10.1016/j.neuron.2017.07.010
- Simon, D., McGeachy, M., Bayır, H. et al. The far-reaching scope of neuroinflammation after traumatic brain injury. Nat Rev Neurol 13, 171–191 (2017). https://doi.org/10.1038/nrneurol.2017.13
- Xiong Y, Mahmood A, Chopp M. Current understanding of neuroinflammation after traumatic brain injury and cell-based therapeutic opportunities. Chin J Traumatol. 2018;21(3):137-151. doi:10.1016/j.cjtee.2018.02.003
- Needham EJ, Helmy A, Zanier ER, Jones JL, Coles AJ, Menon DK. The immunological response to traumatic brain injury. J Neuroimmunol. 2019;332:112-125. doi:10.1016/j.jneuroim.2019.04.005
- Puntambekar SS, Saber M, Lamb BT, Kokiko-Cochran ON. Cellular players that shape evolving pathology and neurodegeneration following traumatic brain injury. Brain Behav Immun. 2018;71:9-17. doi:10.1016/j.bbi.2018.03.033